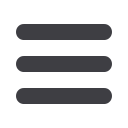
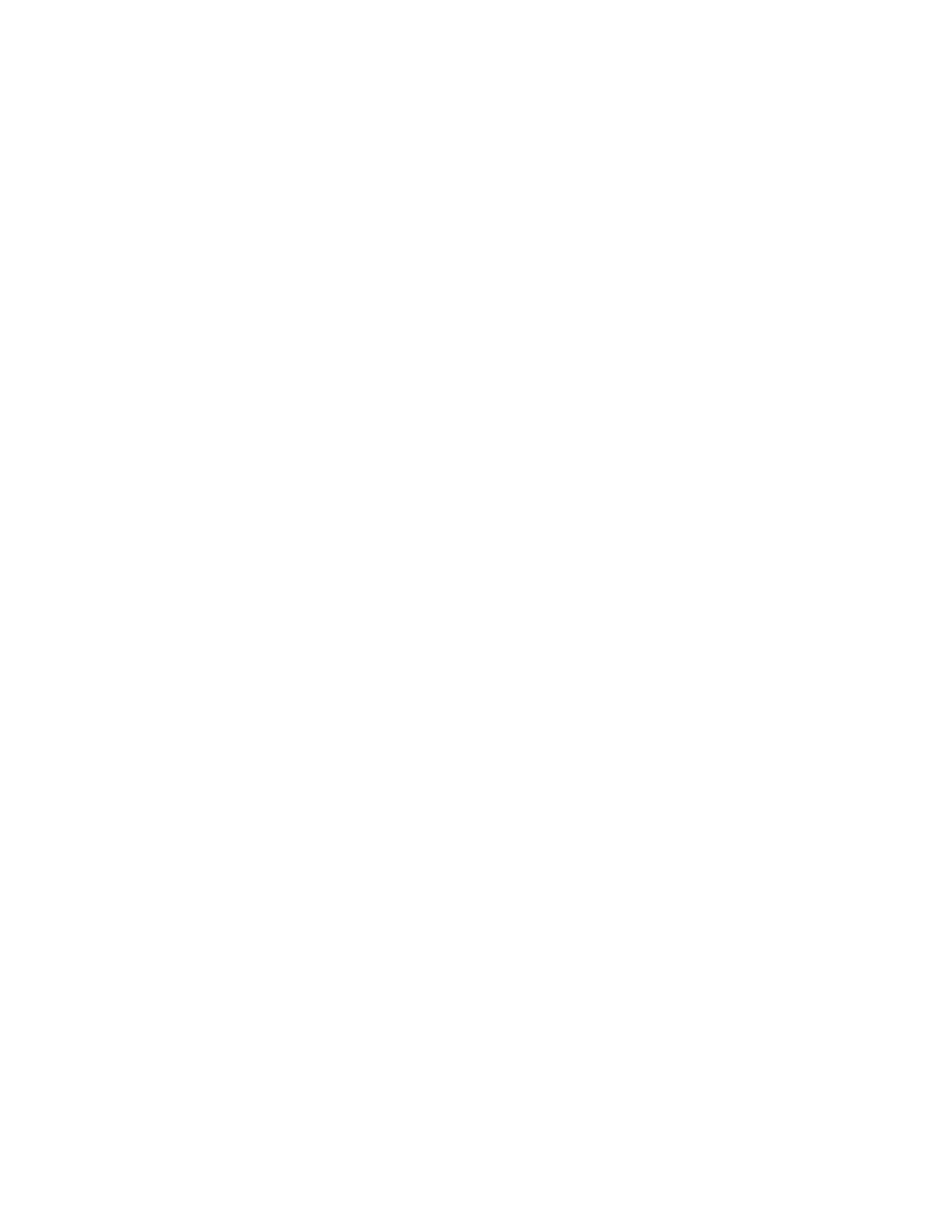
Sunburst Sensors Response, January 12, 2015
General comments:
We thank the ACT personnel and the scientists who participated in the analysis of
quality control samples for their dedication to this important project. Establishing methods to accurately
measure ocean pH and to determine precision and accuracy between methods is important in studying the
effects of climate change and acidification on marine and freshwater environments.
These tests were conducted in laboratory and field settings with a wide range of temperature, salinity, and
pH. These varying conditions test the limits of the instruments, which are generally designed and
optimized for more limited conditions. The SAMI-‐pH was designed to measure pH in the range of 7 -‐ 9 at
salinity 25 -‐ 40, and temperatures 0°C -‐ 40°C. These tests were an opportunity for additional rigorous field-‐
testing of the SAMI-‐pH under more variable conditions. We were also able to test a prototype SAMI-‐pH unit
with Phenol Red (PR) indicator in the freshwater deployment, as this indicator equilibrium range is more
appropriate for freshwater pH.
Lab tests allowed collection of QC samples under static pH/salinity/temperature conditions, whereas in the
field studies the diurnal pH signal ranged from ~0.1 (MLML) to ~0.5 (GERL). When pH is changing rapidly
as in the GERL study, temporal and spatial differences between sensors and collection of QC samples can
cause scatter between the methods. Temperature differences between sensors can have a significant effect
on pH as well, because QC samples are measured at a constant temperature and corrected to the
temperature of the in situ sensors. Considering the challenges, ACT personnel did an excellent job
providing both controlled and field testing conditions representing fresh, brackish, and sea water at
various temperatures and pH, and collecting and analyzing QC samples for the tests. The comparisons
between QC samples and the SAMI-‐pH are addressed below.
Laboratory Test.
Overall, the SAMI-‐pH had excellent performance, operating through 10 weeks of testing
and producing 3140 out of 3154 possible measurements. The overall correlation was excellent and offsets
between SAMI-‐pH and QC pH were good with the largest offsets being in freshwater (Figure 7). The offset
originates from the equilibrium constant for the indicator mCP, which is not well known at low salinity, and
the precision between pH measurements with mCP and the QC method (Phenol Red) are not well
characterized. Bubbles that enter the optical path also likely caused scatter in the SAMI data as shown in
Figures 4-‐6 where significant deviations from the pH baseline are evident. The SAMI-‐pHs are normally
deployed > 3 m depth where bubbles are not significant. This study also provides us valuable data from
which to develop better software for filtering outliers from field data.
Field Tests.
The SAMI-‐pH produced excellent field results operating well through 22.5 of 24 weeks and
collecting 10,483 useable measurements out of 11,375 possible. The SAMI-‐pH correlates well with the QC
pH in all tests except for Moss Landing, where pH scatter later in the deployment, from a high particulate
sample, skewed the data. The SAMI pH difference from the QC pH is excellent at salinity = 35 (Hawaii),
since this is the condition that the instrument is designed for, and also very good in the other conditions
tested. In freshwater, our experimental Phenol Red SAMI-‐pH performed better than the mCP SAMI, as
expected, because the PR equilibrium constant is more appropriate for freshwater pH and the QC method
uses PR. The SAMI-‐pH did not experience pH drift through deployments as long as 3 months, and pH
measurements were not affected by biofouling. Each field test is discussed separately below. The only
general concern in these studies was the observed temperature differences between sensors (See Figure
A). In each test, two temperature sensors were deployed and differences between the two were recorded
(e.g. Figure 8). Temperature differences of > 1°C were seen in some field studies, which would cause errors
in the reported pH at in situ conditions of >0.015, after correction from the QC measurement to the in situ
conditions. Moreover, these temperature differences also likely correspond to small scale pH (DIC and
Alkalinity) spatial gradients making sampling very challenging in these environments. Although these
temperature differences were dismissed as insignificant in the report, in fact, they could contribute
significantly to the apparent accuracy and precision of the deployed instruments.
62